Tissue Engineering: Advances and Innovations in Regenerative Medicine
Fundamentals of Tissue Engineering
Tissue engineering stands at the crossroads of numerous scientific fields, fostering a multidisciplinary approach to develop functional biological substitutes. This section elaborates on the foundational aspects that underpin tissue engineering.
Overview of Tissue Engineering
Tissue engineering is an interdisciplinary field that applies the principles of engineering and life sciences toward the development of biological substitutes that restore, maintain, or improve tissue function. This field incorporates strategies involving the use of cells, engineering methods, and biochemical factors to construct tissues that can often mimic those found in the human body.
Key Concepts in Tissue Engineering
The core concepts in tissue engineering include understanding cell behavior, the role of the extracellular matrix (ECM), and the interaction of cells with scaffolds and biomaterials. Scaffolds are structured materials that house cells, guiding their growth and organization into functional tissues. These materials can be composed of a range of substances including collagen or biodegradable polymers. Biomaterials used in scaffolds must be chosen for their biocompatibility and ability to mimic the natural ECM, which offers both physical and biochemical cues to cells.
Tissue Engineering Strategies
There are numerous strategies in tissue engineering, such as seeded scaffold techniques, where cells are placed on or within a scaffold to create tissue; 3D bioprinting, which involves layer-by-layer precise placement of biological materials, cells and supporting components to generate tissue constructs; and decullarization, where cells are removed from a donor organ, leaving behind an ECM scaffold that can be repopulated with a recipient's own cells. The choice of strategy depends on the specific type and function of tissue to be engineered.
Cell Sources for Tissue Engineering
Cells are the fundamental units in tissue engineering and can be sourced from autologous, allogeneic, or xenogeneic origins. Mesenchymal stem cells are commonly used because of their ability to differentiate into a variety of cell types. Selecting the appropriate cell source hinges on factors such as availability, potential for cell proliferation, and compatibility with the tissue engineering application. Apart from living cells, biologically active molecules like growth factors can be used to stimulate cell growth and differentiation.
Tissue Engineering Applications and Techniques
Tissue engineering merges the principles of biology and engineering to develop tissue substitutes that repair, maintain, or improve the function of damaged human tissues and organs. This field employs a variety of methods and materials to address complex medical challenges.
Repair and Regeneration of Damaged Tissue
Tissue engineering has made significant progress in repairing and regenerating damaged tissues. This includes tissues of the heart, bone, and cartilage, which are critical to the human body's functionality. Approaches include combining scaffolds, cells (such as stem cells and progenitor cells), and bioactive molecules to facilitate in vivo regeneration. For instance, bone marrow-derived mesenchymal stem cells are used to repair bone defects, fostered by the development of new blood vessels (vascularization) and cell adhesion techniques. Differentiation of cells is controlled to ensure that they develop into the desired tissue type.
Drug Delivery and Organoid Systems
Advancements in drug delivery and organoid systems have facilitated in vitro models for testing therapeutic strategies. Organoids are miniature, simplified versions of organs that mimic their complexity and functions. These systems, known as organs-on-a-chip, have revolutionized medical research by providing a more precise model for human physiology. They are used for studying drug effects and disease progression, allowing for more confident clinical translation. Hydrogels and nanomedicine approaches are often integrated with these systems for targeted drug delivery, enhancing the potential for personalized medicine.
3D Bioprinting in Tissue Engineering
3D bioprinting is at the forefront of tissue engineering technologies. Using a layer-by-layer method, this technique allows for the precise placement of cells and biomaterials to create complex tissue structures. 3D bioprinting has the potential to fabricate bioartificial tissues with intricate architectures, like vascular networks within engineered tissues. Pioneers such as Langer and Vacanti have noted its potential to not only regenerate tissues but also to help in creating artificial tissues for transplantation, pushing the boundaries beyond traditional sutures and surgical repairs. The integration of computational modeling and artificial intelligence has greatly improved the design and functionality of 3D-bioprinted tissues.
Challenges and the Future of Tissue Engineering
Tissue engineering stands at the forefront of regenerative medicine, aiming to repair or replace damaged organs and tissues. While progress has been significant, the field faces several clinical challenges, and emerging trends hint at an innovative future.
Clinical Challenges in Tissue Engineering
The path to clinical application of tissue engineering is hindered by complex biological challenges. One primary issue is securing dependable sources of cells that can repopulate and regenerate tissues. For instance, bone marrow-derived mesenchymal stem cells present a promising cell source due to their plasticity and ability to differentiate. However, the efficient expansion and maintenance of these cells' potency remains a critical concern.
Finding optimal biomaterials is also crucial; the ideal material should exhibit properties such as biocompatibility, biodegradability, and the ability to promote cell adhesion and proliferation. Collagen, for instance, has been widely used due to its natural occurrence in the human body and its role in providing structural support. Yet, its mechanical properties and immunogenicity need to be tightly controlled.
In terms of organ repair, creating complex structures like vascularized tissues that can integrate seamlessly with the human circulatory system poses significant challenges. Vascularization is critical for delivering nutrients and oxygen to engineered tissues, thus ensuring the survival of transplanted cells. Additionally, innervation within bioengineered tissues is essential for restoring full functionality, particularly in tissues like skin, muscle, and organs.
Another hurdle is the immunogenicity of tissue-engineered scaffolds, which must be minimized to prevent rejection. The development of tailored bioinks for 3D bioprinting aligns with this need, offering a platform for creating patient-specific tissues with reduced immune responses.
Innovations and Emerging Trends
Despite the challenges, the future of tissue engineering is bright with emerging trends and innovations. Advanced 3D bioprinting technologies are revolutionizing the way artificial tissues are created, allowing for the precise layering of cells and biomaterials to form complex, functional structures like heart tissue or vascular networks.
Organoids and artificial tissues are being developed as platforms for drug delivery systems, aiding in personalized medicine by testing drugs on bioengineered tissues that mirror the patient’s own cells. This aligns closely with the goals of regenerative medicine, shifting the paradigm from treating symptoms to repairing the underlying issue.
Cell therapies are becoming more sophisticated, with an emphasis on understanding and guiding cell behavior through computational models. This is where artificial intelligence steps in, offering predictive models for cell proliferation and differentiation that can drastically reduce the time and cost associated with experimental research.
Regarding clinical translation, the integration of drug delivery mechanisms within tissue-engineered constructs is enhancing the therapeutic potential of these systems, providing localized, controlled release of medicinal agents directly where needed, thus maximizing efficacy and reducing systemic side effects.
In conclusion, the field of tissue engineering is rapidly evolving, with ongoing research addressing current challenges and advancements in technology paving the way for future breakthroughs. The combination of a deeper understanding of cell behavior, advancements in biomaterials, and innovative biofabrication techniques contribute to the accelerated progress towards successful clinical applications.
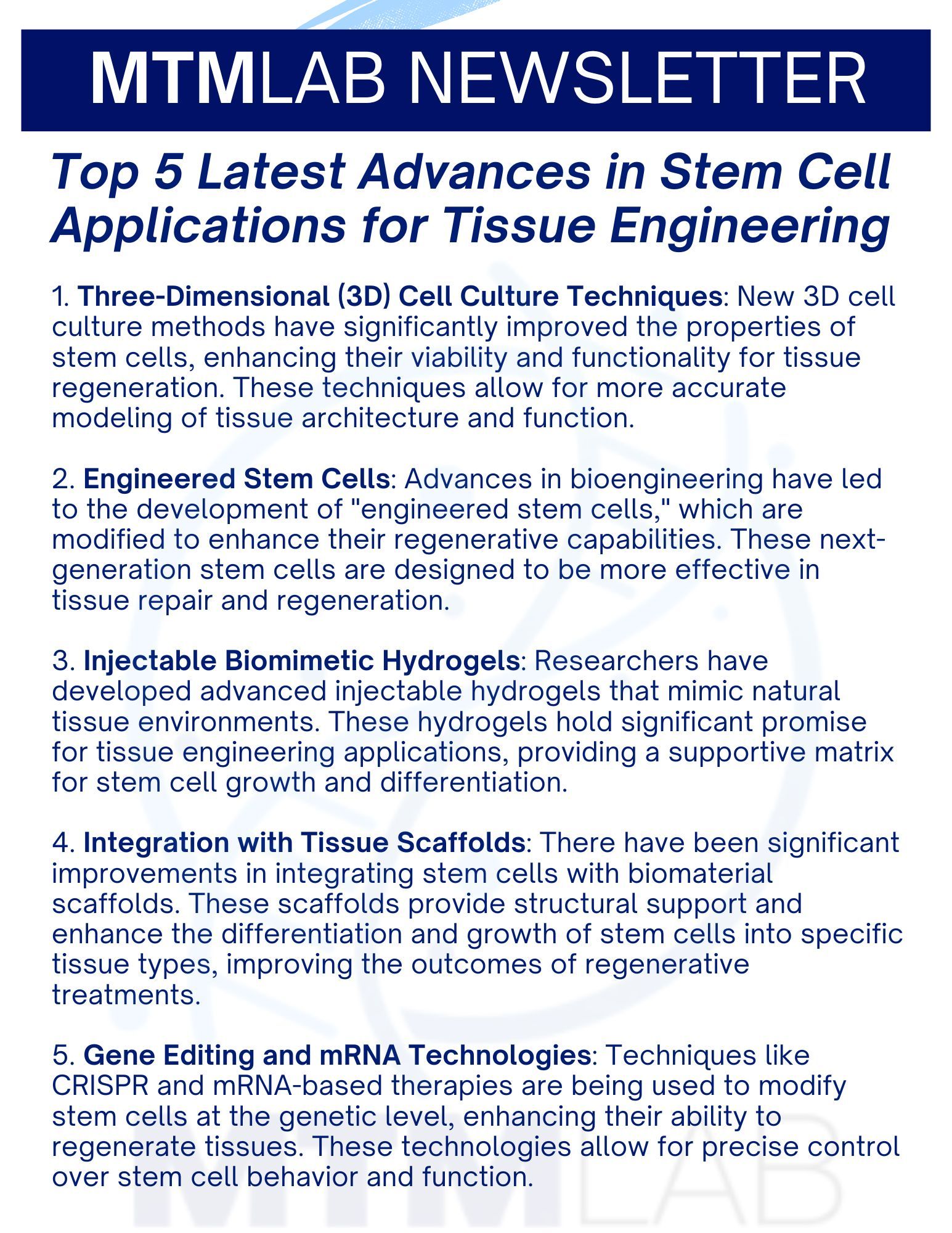
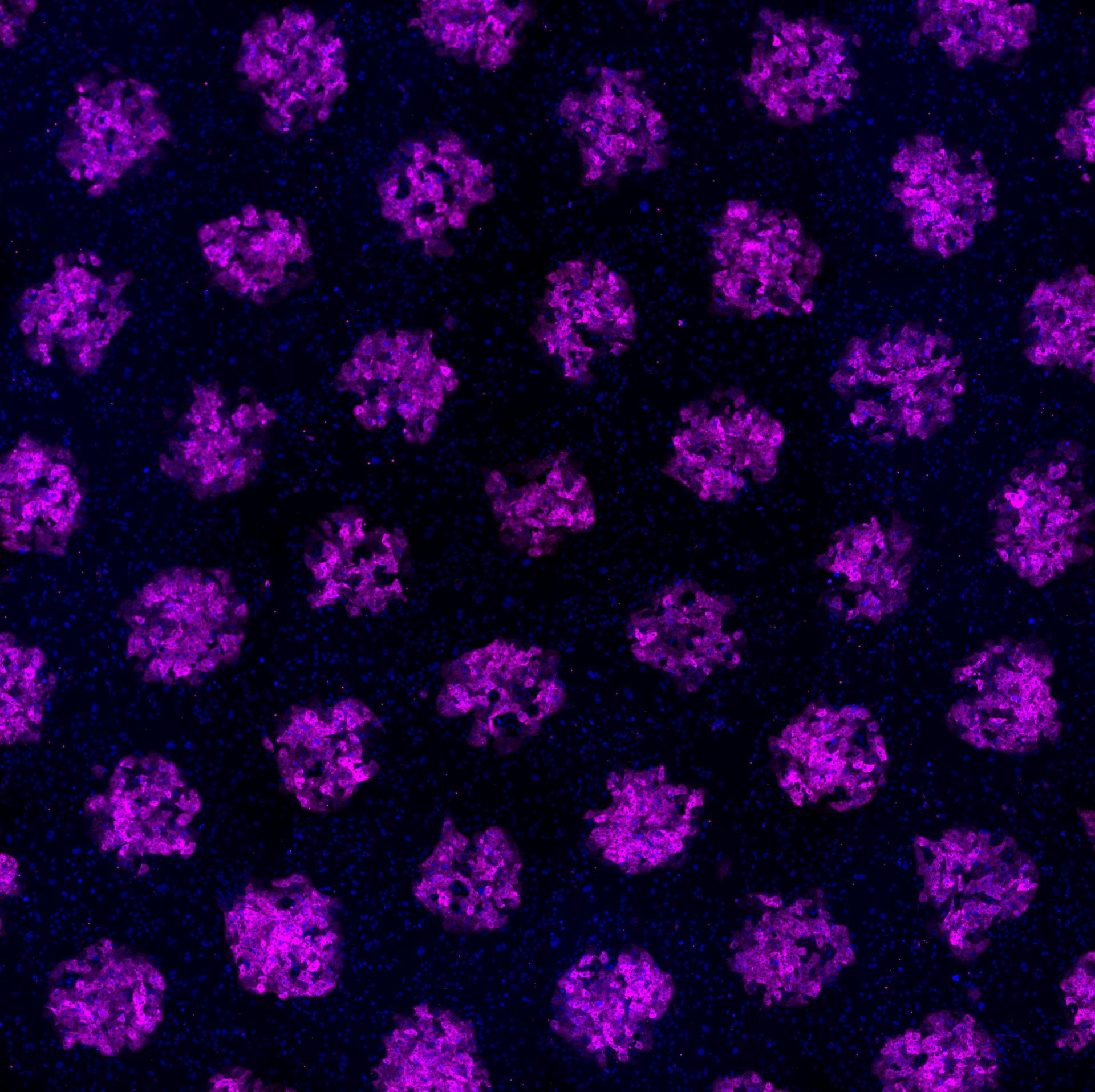