Biomaterials for Regenerative Medicine: Innovations and Applications
Fundamentals of Biomaterials for Regenerative Medicine
Biomaterials are pivotal in regenerative medicine, facilitating the restoration of tissue function by supporting the integration and growth of new cells. Their selection and application are grounded in biomaterials science and biomedical engineering principles.
Overview of Biomaterials
Biomaterials are materials engineered to interact with biological systems for a medical purpose, be it diagnostic or therapeutic. They encompass a wide range of substances, from metals to polymers and ceramics. In the context of regenerative medicine, these materials are designed to mimic the extracellular matrix, providing a scaffold for tissue formation. A biomaterial scaffold is essential for supporting cellular attachment, proliferation, and differentiation.
Classification and Selection of Biomaterials
Biomaterials are generally classified into four primary types:
- Metals: Used for their strength and durability but limited due to potential corrosion.
- Ceramics: Biocompatible and often used for bone tissue engineering.
- Degradable Polymers: Break down over time making them suitable for temporary scaffolds.
- Nondegradable Polymers: Long-lasting and used for permanent implants.
- Hydrogels: Highly hydrophilic and can mimic the natural tissue environment.
The selection of a particular biomaterial for regenerative medicine is based on:
- Biocompatibility
- Biodegradability
- Mechanical properties
- Physical characteristics
Regenerative Medicine: A Primer
Regenerative medicine is an interdisciplinary field that applies the principles of engineering and life sciences toward the repair, replacement, or regeneration of tissues or organs to restore or establish normal function. It relies heavily on the use of biomaterials to create environments conducive to cell growth and differentiation. Regenerative strategies include cell-based therapies, tissue-engineered organs, and the application of biomaterial scaffolds as templates for tissue regeneration.
Design and Fabrication Approaches
The creation of biomaterials for regenerative medicine involves sophisticated techniques to fabricate scaffolds that support tissue development. The methods must ensure biocompatibility and the appropriate microenvironment for cell growth and differentiation.
Scaffold Fabrication Techniques
Scaffold fabrication has to meet specific criteria depending on the application. Bone tissue engineering, for example, often employs scaffolds made of materials such as hydroxyapatite or tricalcium phosphate because of their osteoconductive properties.
- Electrospinning: used to create nanofibers that mimic the extracellular matrix, suitable for soft tissue engineering.
- 3D Printing: allows for the precise construction of scaffolds with customized shapes and sizes, beneficial for bone and cartilage tissue engineering.
- Solvent Casting and Particulate Leaching: often used with alginate or chitosan, providing porous scaffolds that encourage cell migration and nutrient transport.
Advances in Hydrogel Technology
Hydrogels stand out due to their high water content and ability to closely resemble living tissue. They are pivotal in scaffold design for their porosity and soft tissue compatibility.
- Synthesis: involves cross-linking hydrophilic polymers; often used hydrogels include those based on polyethylene glycol.
- Tailoring Properties: modifications such as incorporation of bioactive signals can enhance cellular responses. Alginate and hyaluronic acid hydrogels have seen extensive use, particularly for cartilage tissue engineering.
Synthetic and Natural Biomaterials
Synthetic polymers like PLGA (poly(lactic-co-glycolic acid)) and natural biomaterials like collagen and silk biomaterials each have unique advantages in regenerative medicine.
- Synthetic polymers: provide controlled degradation rates and mechanical strength, with customization possibilities for specific tissue targets.
- Natural biomaterials: Silk and collagen are widely used for their exceptional mechanical properties and biocompatibility.
- Functional and Novel Biomaterials: Efforts in synthesis have led to novel biomaterials that can respond to biological stimuli or incorporate inorganic components, including biopolymer-based inorganic biomaterials like bioactive glasses.
Clinical Applications and Challenges
In the arena of regenerative medicine, clinical applications of biomaterials confront various challenges, including ensuring tissue compatibility, optimising mechanical properties, and regulating stem cell fate.
Biomaterials in Tissue Regeneration
The use of biomaterials like decellularized extracellular matrix (ECM) and hyaluronic acid has showcased potential in tissue regeneration applications. Bone repair, for instance, leverages osteoconductive scaffolds to support osteogenic differentiation. Conversely, in nerve regeneration, bioactive scaffolds aim to facilitate directional growth. The efficacy of these materials is afflicted by their mechanical properties and their ability to integrate with host tissue and induce neovascularization.
Stem Cells and Regenerative Medicine
Incorporation of stem cells, including mesenchymal stem cells (MSCs), embryonic stem cells, and induced pluripotent stem cells (iPSCs), into biomaterial constructs offers a powerful avenue for regenerative medicine. These cells can be directed towards specific lineages, such as chondrogenic differentiation for cartilage repair or progenitor cells for cardiac tissue repair. Integrating scaffolds with stem cell sheets has been a novel approach to address complex diseases and trauma.
Biomedical Engineering in Clinical Settings
Biomedical engineering intersects with clinical practice in orthopedics and organ regeneration, among others. For instance, the Mayo Clinic's Biomaterials and Regenerative Medicine Laboratory investigates biomaterials for bone grafts. A significant hurdle is the adaption of drug delivery systems to regulate inflammation and healing, with a current focus on modulating immune responses, such as macrophage behavior, in the regenerative processes.
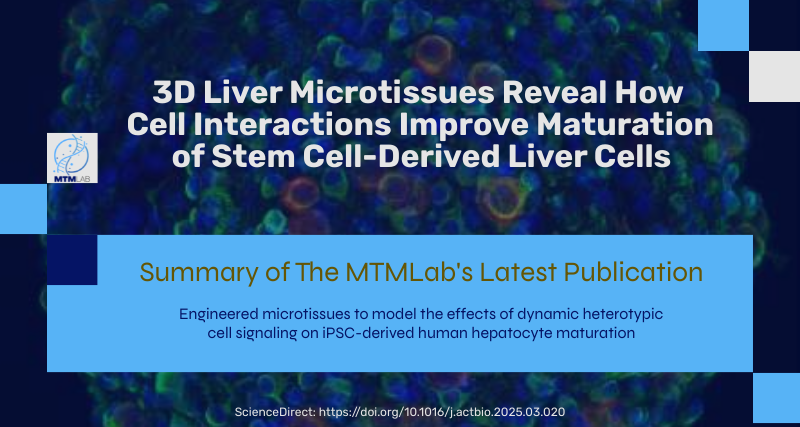
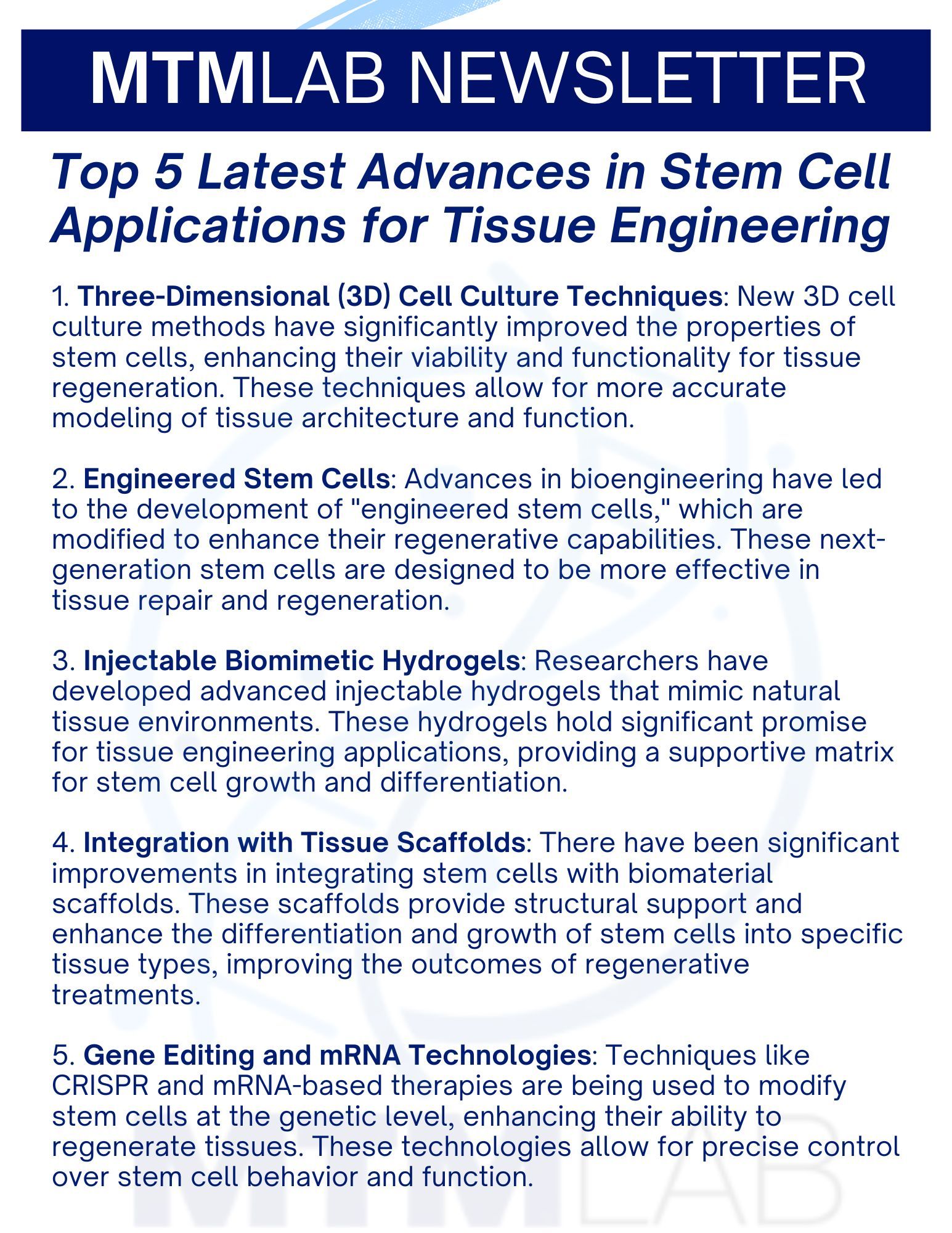